Sorry, this entry is only available in Español.
Tag Archives: solar energy in latin america
Mexico Solar Thermal
As in most Latin American countries, in Mexico, statistics in solar thermal energy field are not up-to-date and renewable energies prospects do not include this source of generation.
In Mexico, in 2010, solar systems to heat water were installed in an equivalent area of 272,580 m2, reaching an accumulated area of 1,665,502 m2.
According to estimates, in next 4 years almost double the production area by solar thermal energy.
Although we do not find data about current installed capacity in Mexico, we can conclude that this type of energy has had a great growth in recent years and that it is likely that the installed capacity has doubled again.
Due to country average radiation levels, a solar thermal installation for domestic hot water has become a very profitable investment in Mexico, since water heating causes the greatest gas consumption and with this application, gas use is reduced by up to 80% in regions with higher radiation.
Recently there has been a noticeable decrease in prices of solar equipment for domestic hot water.
The factors that allow this to happen are imports, easy manufacturing, technology maturity and competitiveness between national and international companies that offer this type of equipment.
In Mexico, there are important companies that manufacture low temperature thermal solar energy equipment. The first began in 1940 in Guadalajara.
Several government programs have promoted low cost acquisition of solar heaters by residents of areas where the gas network does not reach.
Other solar thermal energy applications that have increased considerably are swimming pools conditioning and water heating for industrial processes.
Hermosillo was one of the first states to adopt this type of technology for industrial processes in Mexico.
A cement company uses a thermal 291 KW equipment to operate a 75 tons of single effect cooling system. This was the first air conditioning system based on renewable energy in Latin America. The parabolic cylindrical collectors are located on the roof and on one side of corporate building; it operates in a range from 70 ° C to 95 ° C.
Other systems have been installed for generating heat purpose.
Mexican companies have commercially developed parabolic-linear solar concentrators to generate thermal energy between 50 ° C and 200 ° C. These systems are used mainly in food sector.
Some of the companies that currently have this alternative energy generation in the country are:
– Food company: installation of 80 solar concentrators for process heat generation and absorption chiller supply.
– Dairy company: installation of 70 solar concentrators for direct heat input in dairy products processing.
– Egg producing company: installation of 80 solar concentrators for boiler preheating.
Despite advances, there is still much room for solar thermal technology development in Mexico.
The final impulse could come from special financing lines implementation, since for a large population sector initial system investment is still very high.
All you need is Sun. All you need is Sopelia.
The Solar Converter
They are equipment capable of altering voltage and characteristics of electric current they receive to transform it into suitable for specific uses.
Those that receive direct current and transform it into direct current with a different voltage are called DC-DC converters. They are not widely used in photovoltaic systems.
Those that receive direct current and transform it into alternating current are called DC-AC converters or inverters. The function of an inverter is to change a DC input voltage to a symmetrical AC output voltage, with the magnitude and frequency desired by user.
They allow to transform 12V or 24V direct current that modules produce and store batteries, in 125V or 220V alternating current.
This allows use of electrical devices designed to work with AC.
A simple inverter consists of an oscillator that controls a transistor, which is used to interrupt incoming current and generate a square wave. This square wave feeds a transformer that softens its shape, making it look like a more sinusoidal wave and producing the necessary output voltaje.
The voltage output waveforms of an ideal inverter should be sinusoidal.
This gives rise to different types of inverters:
1) Square wave inverters: they are cheaper, but less efficient. They produce too many harmonics that generate interference (noise). They are not suitable for induction motors.
Recommended if you want AC power only for a TV, a computer or a small electrical device. Inverter power will depend on device nominal power (for a 19 ” TV a 200 W inverter is enough).
2) Modified sine wave inverters: they are more sophisticated and expensive. They use pulse width modulation techniques.
Wave width is modified to bring it as close as possible to a sine wave. Harmonics content is less than in square wave.
They are the ones that offer best quality / price ratio for lighting, television or frequency inverters connection.
3) Pure sine wave inverters: with a more elaborate electronics, a pure sine wave can be achieved.
Until recently these investors were large, expensive and inefficient; but lately, has been developed equipment with 90% or more efficiency, telecontrol, energy consumption measurement and battery selection.
Since only induction motors and most sophisticated devices or loads require a pure sine wave form, it is usually preferable to use modified sine wave inverters; which are cheaper.
Inverters must be dimensioned from two variables.
First is considering electrical power wattages that inverter can continuously supply during its normal operation.
Inverters are less efficient when used at a low percentage of their capacity. For this reason it is not advisable to oversize them and they must be chosen with a power as close as possible to that of load consumption.
Second is starting power. Some inverters can supply more than their nominal capacity for short time periods. This capacity is important when using motors or other loads that require 2 to 7 times more power to start than to stay running once they have started (induction motors, high power lamps).
Incorporating an inverter is not always the best option from energy efficiency point of view. It may seem an easy solution to convert all solar system output to a standard AC power but it has several disadvantages.
First is that it increases system cost and complexity.
An inverter also consumes energy (in addition to 15% for performance loss) and therefore decreases overall system efficiency.
For a small house electrification (light points, TV and a small appliance) it is possible and profitable to do without the inverter.
For lighting it is better to invest in low voltage lights instead of investing in an inverter.
Laying of 2 lines can be interesting: one connected to batteries to feed points of low consumption lighting or LED and devices that consume DC and another connected to inverter to power appliances that consume AC.
The advantage of the inverter is that operating voltage is much higher and therefore the use of thick cables can be avoided. Especially when wiring is extremely long it may be economically feasible to use an inverter.
A feature that incorporates most modern converters is possibility of operating as battery chargers, taking alternating current from a generator or grid.
This is an extract of contents included in Technical-Commercial Photovoltaic Solar Energy Manual and Sopelia e-learning training.
All you need is Sun. All you need is Sopelia.
Solar Collectors Clamping And Anchoring
Proposed solution must comply, in order of importance:
– That it’s enough safe .
– That its cost be as low as possible.
– Speed and simplicity in assembly.
A method currently used is anchoring by chemical plug.
There are structures are of different materials. The most commonly used are aluminum and stainless steel.
Manufacturers usually sell the collector with its structure, although you can always design your own structure.
It is not advisable to transfer building cover with the anchor (it can cause leaks).
In case of large installations, a pre-assembly work can be carried out to make assembly on roof faster and cheaper.
In near coast areas, structure must be hot dip galvanized.
Screws should be made of stainless steel or corrosion resistant material.
Anchoring type will be based on:
1) Wind forces that must endure. If collector is South oriented (we are in the Northern Hemisphere), wind that represents a risk is that coming from North (it is the inverse if we are in the Southern Hemisphere), which will exert tensile force on the anchors. The South wind will exert compressive force, not so dangerous. Wind force on a surface is:
f = P. S. sen2α
f = Weight to counteract wind strength.
P = wind load (Kg / m2).
S = collector surface (m2).
sin2α = angle of inclination sine.
Wind force is decomposed into f1, which incites perpendicularly to collector surface and in f2, which does it in parallel.
f1 force is at the end what counts and what is obtained from previous formula.
2) Collectors orientation and inclination. Collectors are oriented towards Ecuador. Normally, if we are in Southern hemisphere, they are oriented towards North and vice versa. Deviations of up to 20% with respect to optimal orientation do not significantly affect system performance and thermal energy contributed.
Collector’s inclination angle will depend on solar equipment use. Orientates inclinations:
• All year use (H.W.S.): inclination angle equal to geographical latitude.
• Winter preferably use (heating): inclination angle equal to geographical latitude + 10º.
• Summer period preferred use (outdoor pools heating): inclination angle equal to geographical latitude – 10º.
Variations of ± 10º with respect to optimum inclination angle practically do not affect performance and useful thermal energy provided by solar equipment.
3) Collecting surface must be free of shadows. In the most unfavorable day of use period, installation must not have more than 5% of useful surface area covered by shadows.
Projected shadows practice determination is made observing environment from collector´s lower edge midpoint, taking the North-South line as a reference.
By making an angular sweep on both sides, we will try to locate nearby obstacles with an angular height greater than 15º / 25º.
A more accurate determination of possible shadows can be made using system sizing software based on simulation methods.
4) Minimum distance between collectors. Separation between collectors rows must be established so that at solar noon of most unfavorable day (minimum solar height) of use period, the shadow of upper edge of a row will be projected, at most, on lower edge of following row.
The formula of minimum distance between collectors is:
DT = L (senα / tan H + cosα)
H is the minimum solar height, which is:
H = (90º – latitude place) – 23.5º
L is collector´s height
If collector’s rows were arranged on a non-horizontal surface, expression would become:
DT = L ((sin (α – β) / tan (H + β) + cos (α – β))
α is still collector inclination angle respect to horizontal.
β is roof inclination angle respect to horizontal. It is positive if cover inclination angle direction coincides with that of collector; and with a negative value otherwise.
5) Finally, calculations must be carried out to ensure that cover or support will be able to support collectors weight, and that of the tank in case of thermosiphon and compact systems.
The R + D + I area of Sopelia has developed Solar Layout, the mobile app that allows collectors and modules to be optimally located at installation site.
All you need is Sun. All you need is Sopelia.
(Español) Energía Solar Dónde Quiera Que Estés
Free Solar Tools (IV)
On Internet we can find free tools for basic or low complexity solar systems dimensioning and for certain components or accessories estimation.
Sopelia research team has carried out an exhaustive search and testing from which a new corporate website section called Free Solar Tools has been created.
Selected tools were classified into 4 categories.
Today we will analyze the fourth of them: Solar Photovoltaic.
In the first category we have already analyzed tools to obtain data about solar resource and other variables to be considered in energy estimation solar system will provide in our location.
In the second category we have analyzed tools to calculate the “load”, ie the energy demand to be met.
In the third category we have analyzed tools for solar thermal systems dimensioning and system accessories estimating.
Now we are going to analyze tools for solar photovoltaic systems dimensioning and to estimate others individual components of a system.
The order of the tools is not random. We have prioritized the most intuitive, the most universal and those that can be used online without download.
For this fourth category our selection is as follows:
1) Solar Calculator
Approximate calculation tool from which budget, production data and system performance study is automatically obtained.
A Navigation Guide and Manuals can be found at page bottom.

2) Off-grid Solar Systems Calculator
Free online application for off-grid solar systems calculation.
It allows users to introduce new components from any manufacturer and product datasheets to be considered in the calculation.
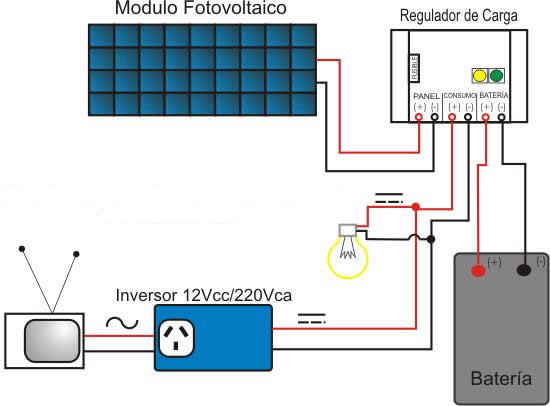
3) Off-grid Systems Scale Calculator
Solar basic estimation of off-grid systems. Solar modules, batteries, controller and inverter calculation.

4) Solar Water Pumping Calculator
Calculator to obtain approximate energy needs figures for solar water pumping.

5) Solar & Wind Energy Systems Calculation
Tool which determines requirements to meet solar and / or wind contribution for electrification and pumping needs.

6) Grid Connected System Online Simulation
Online application to estimate production and economic income of a grid-connected system.

7) Battery Bank Capacity Calculator
Calculator to estimate battery bank size needed to keep consumption by solar operation.

8) Wire Section Calculator
Tool in JavaScript format for copper and aluminum DC wire calculation.
All you need is Sun. All you need is Sopelia.
The Solar Charge Controller
Charge controller is a device located between photovoltaic modules and batteries as an element of an isolated solar system.
Modules output voltage is set some volts higher than voltage battery needs to charge. The reason is to ensure that modules will always be able to charge the battery, even when cell temperature is high and generated voltage decreases.
This causes the drawback that once battery reaches its full charge state, module continues to try to inject energy producing an overload that, if not avoided, can destroy battery.
Charge controller is responsible for extending batteries life protecting them from overload situations, controlling load phases depending on their status and even reaching the cut depending on load needs of them.
Charge controllers may be working in one of the following situations:
– Equalization status: equalization of batteries charge, after a period of low charge.
– Deep charging state: regulation system allows charging until reaching final load voltage point.
– Float state: battery has reached a charge level close to 90% of its capacity.
– State of final charge and flotation: regulation system zone of action within Dynamic Flotation Band (range between final load voltage and nominal voltage + 10%).
To know which regulator to incorporate into a photovoltaic system it is necessary to know some elementary parameters.
First, one is isolated solar system nominal voltage. Batteries voltage and photovoltaic solar field define this voltage. Typical values are 12, 24, 48 and up to 60 volts.
The other parameter is photovoltaic modules system load current. It is recommended to multiply short circuit current Isc under standard conditions by 1.25 so that charge controller is always able to withstand current produced by modules.
Known system voltage and determined current value, we can choose the right charge controller. If there are still doubts, we can consult with provider technical department.
The simplest design is one that involves a single stage of control. Charge controller constantly monitors battery voltage but controls charge or discharge, never both. They are the cheapest and the simplest.
This can be achieved by opening the circuit between photovoltaic modules and battery (serial control) or by short-circuiting photovoltaic modules (shunt control).
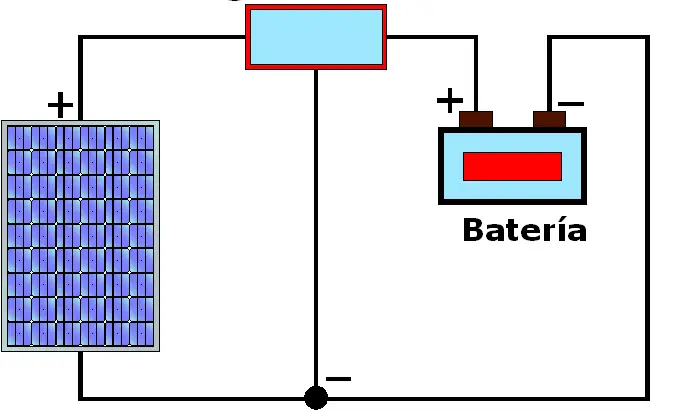
In case of controllers that operate in two control stages, the two functions are controlled, both charge and discharge of battery. They are more expensive, but they are the most used.
Current charge controllers introduce microcontrollers and control 3 and up to 4 control stages.
During last years a new generation of charge controllers has been developed, whose main characteristics are to make photovoltaic field work at maximum working point and to always render it optimally.
These charge controllers are known as power maximizers or MPPT.
Another advantage of these devices compared to conventional controllers is the possibility of working with a different voltage in the generator field (solar panels) and batteries.
This directly influences in being able to put several modules in series, elevating system tension.
Working with lower currents we can reduce considerably voltage drop losses and use smaller cable sections and therefore of lower price.
For the choice of a conventional controller or an MPPT, we have to assess cost overruns that these systems have compared to benefits that it gives us due to system performance increase. In some cases, annual power increase can reach up to 30% compared to conventional controller.

Charge controller may not be essential in installations where the ratio between modules power and battery capacity is very small (eg: oversized batteries for safety reasons) so that charging current can hardly damage battery.
If modules field power in W is less than 1/100 battery capacity in W / h, charge controller may not be incorporated.
It can also be dispensed with a charge controller if system has self-regulated solar modules (not recommended for extreme climates).
This is an extract of contents included in Technical-Commercial Photovoltaic Solar Energy Manual and Sopelia e-learning training.
All you need is Sun. All you need is Sopelia.
Free Solar Tools (III)
On Internet we can find free tools for basic or low complexity solar systems dimensioning and for certain components or accessories estimation.
Sopelia research team has carried out an exhaustive search and testing from which a new corporate website section called Free Solar Tools has been created.
Selected tools were classified into 4 categories.
Today we will analyze the third of them: Solar Thermal.
In first category we have already analyzed tools to obtain data about solar resource and other variables to be considered in energy estimation solar system will provide in our location.
In the second category we have analyzed tools to calculate the “load”, ie the energy demand to be met.
Now we are going to analyze tools to solar thermal system dimensioning and others to estimate individual components of a system.
The order of the tools is not random. We have prioritized the most intuitive, the most universal and those that can be used online without download.
For this third category our selection is as follows:
1) Solar Thermal Calculator
Approximate calculation tool from which budget, production data and system performance study is automatically obtained.
A Navigation Guide and Manuals can be found at page bottom.

2) Simulation for Solar Thermal System Pre-design
Online application based on the TSOL software that allows solar energy system simulating to ACS and ACS + heating contribute.
Available in German, English, Spanish and French.
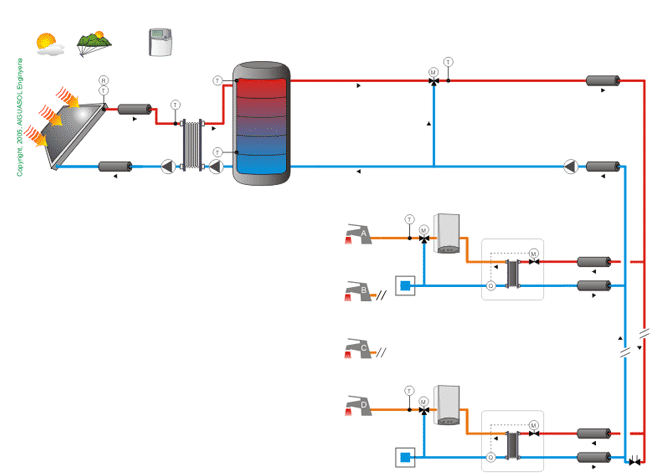
3) Solar Fraction Calculation
Free download program developed by IDAE (Institute for Energy Diversification and Saving) and ASIT (Solar Thermal Industry Association) that allows to define a wide variety of solar systems introducing a minimum of project parameters, associated to each system configuration; and in this way, obtain solar system coverage on ACS and pool conditioning energy demand.
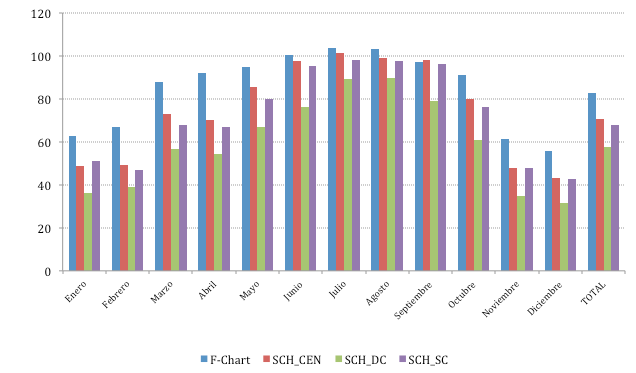
4) Solar Expansion Vessel Calculation
Tool developed to calculate solar expansion vessel volume.
Volume values (total circuit, solar collectors, pipes), Maximum system temperature (ºC), Glycol concentration (%), Height between expansion vessel and system highest point (minimum value 1 Bar) and safety valve Pressure setting must be introduced.
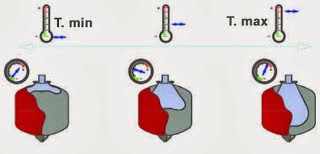
5) Thickness Insulation Pipes Calculation
Calculator that allows to estimate minimum and more economical water pipes insulation thickness.
Pipe Grade and Size, Insulation Material, Humidity and Temperature (Internal and Ambient) must be entered.

All you need is Sun. All you need is Sopelia.
Other Thermal Collectors
We have already talked about flat solar collectors and vacuum tube collectors.
Air collectors are also found in collectors without concentration category.
They are flat and their main characteristic is to have air as heat transfer fluid.
They do not have a maximum limit temperature (convective processes have less influence on the air) and work better in normal circulation conditions, but in contrast they have a low heat capacity and heat transfer process between plate and fluid is not good.
Its main application is heating.
Externally it is not possible to distinguish an air collector from a water collector. It is in the absorber where greatest differences are found. It has a rough shape and lacks the classic pipe of water collector ducts. The air circulates freely on absorber surface collecting the heat that it transforms.
Being a technology that has not been widely disseminated until now, there is no standardized solar collector model and each manufacturer makes its own model.

There are also conical or spherical thermal solar collectors.
Their main characteristic is that they simultaneously constitute collection and storage unit.
Its catchment surface is conical or spherical with a same geometry glass cover. With this form it is achieved that illuminated surface throughout the day, in shade absence, is constant.
Their installation is simple, but they present water stratification problems and useful catchment surface is small.
Its main application is sanitary hot water in single-family homes production and in very benign climates, since large storage surface, weather exposed, causes great energy losses.
Finally, in collectors without concentration category, we find outdoor swimming pools solar collectors.
They are made of rubber, polypropylene or polyethylene; and incorporate in their manufacturing process substances that protect them from plastics natural tendency to degrade under ultraviolet rays action.
They also carry other additives to protect them from chemical agents used in pool water purification. They have an acceptable night frosts resistance.
They are used mainly to heat pools water and thus be able to prolong its use for several more months.
These collectors do not have cover, neither with housing nor with insulating material. They are constituted by naked plate collector. This is because working temperature will not exceed 30ºC in any case and at this low temperature, radiation and conduction losses are very small, making it possible to dispense with covers and insulation.
It is not necessary to use any type of heat exchanger or accumulator, because pool water flows directly through collectors.
They need a frame because they are usually not rigid, but they can also be placed directly on a roof, or even on the ground. By being flexible, absorb surface irregularities on which they rest.
These equipment enjoy an approximate 10 years lifespan. They need little maintenance and there is little risk of corrosion, as they are synthetic.
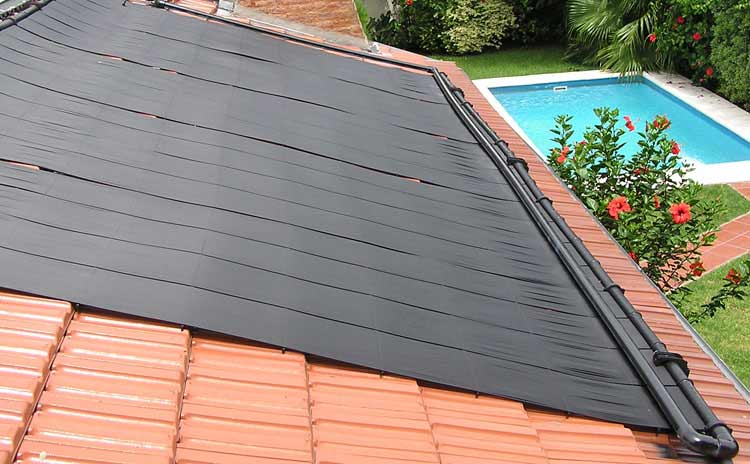
The second major group is that of solar collectors with concentration.
Its most common use is not at domestic level but in thermoelectric plants and facilities that work at medium and high temperature.
These collectors concentrate the solar radiation received in a very small surface receiving element (a point, a line).
Being smallest receiver and concentrated radiation, it allows a better solar energy absorption.
They are capable of providing temperatures above 300 ° C with good yields.
Concentration collector plants generate high temperature steam for industrial processes and to produce electricity.
There are concentration collectors of various types (tower, cylindrical-parabolic, Stirling engine).
Nevertheless, all of them have in common that they demand to be equipped, to be efficient, with a tracking system that allows them to remain constantly located in best position to receive the Sun’s rays throughout the day.
One of the drawbacks of most concentration collectors (and especially the cylindrical-parabolic) is that they only take advantage of Sun direct radiation, that is, they only take advantage of solar rays that actually hit their surface. They are not able, on contrary, to capture diffuse solar radiation.
Therefore, they are not convenient in climatic zones that, although they receive an acceptable solar radiation amount, are relatively cloudy. They are only effective in authentically sunny áreas.
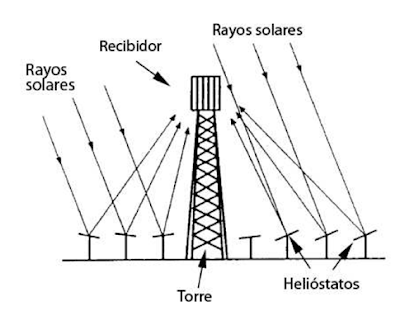
This content was extracted from the Solar Thermal Energy Technical-Commercial Manual and is part of Solar e-learning.
All you need is Sun. All you need is Sopelia.
Honduras Solar PV
Honduras is a country where news about massacres, multiple forms of violence, corruption, instability and political intrigues usually comes out and in which two thirds of its 8 million inhabitants live in poverty while the 10% who receive higher salaries, accounting for 42% of national income and poorest 10% only receives 0.17%.
However, there is a sector in which Honduras stands out at regional level: renewable energies and, especially, solar energy.
Honduran government introduced fiscal incentives for photovoltaic installations in 2013.
A tariff supplement for first 300 PV MW that entered into operation before August 1, 2015 was also approved.
In 2015, Honduras and Chile were the largest PV markets in Latin America.
At the end of 2017, total private capital investment for PV plants construction exceeded US$ 1,600 million.
Investment has been divided into 12 solar plants that are already operational and add up to 405 MW; 39% of country’s private sector renewable capacity, amounting to 1,047.07 MW.
In general calculation, 61% of country’s energy comes from renewables, and in 2017 it became the first country in the world with 10% solar energy in its electric mix.

Most emblematic project is Nacaome-Valle Solar Park, which generates the energy consumed by some 150,000 Honduran families every day.
It has 480,480 modules with capacity to produce up to 125 MW of alternating current (AC) peak power.
It took more than 1,000 material containers, US$ 240 million in investment and the help of more than 1,200 employees who changed shifts without stopping, to build and start operating the plant in less than 2 years.
Photovoltaic modules receive the radiation to generate between 600 and 850 V, in CC form. With use of inverters, this energy is converted into AC, which passes through transformers to raise its Voltage to 34.5 kV and distribute it around the park.
Finally, this current is transmitted to plant electrical substation, where voltage rises to 230 kV to be transmitted throughout the country by Central American Electric Network, which arrives from El Salvador, passes through Honduras and goes to Nicaragua. .
The Nacaome solar plant has been an engine of economic, scientific and academic development for Honduras people and a monumental engineering work that has put Central American nation on sustainable energy industry international map.
Construction of Los Prados solar park, which would have 53 MW and should have started operations at end of 2016, is being halted by local residents protests who fear possible damage to their people caused by the park.
A solution is currently being sought between authorities and settlers, since everything is ready for work execution, but news is not encouraging.
All you need is Sun. All you need is Sopelia.